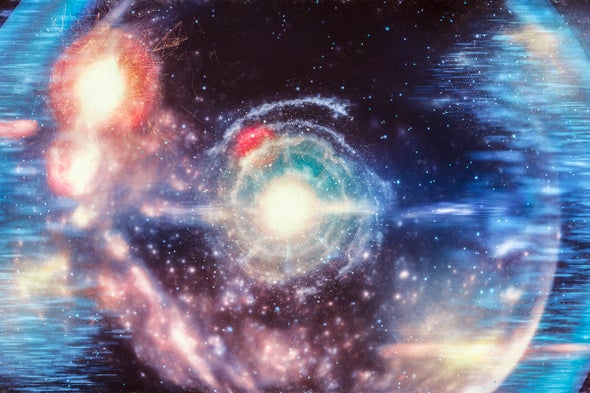
In this age of big science, with fundamental physics generally tested in sprawling laboratories such as CERN and LIGO, maverick individuals who make a big difference through their spot-on hunches are an increasingly rare breed. Those who did so in the past, therefore, have assumed an almost mythic quality. Their excellent guesses changed history in a way that would be much harder today because of the complexity of many areas of research, often requiring massive collaborations.
It has been 20 years since the death, on August 20, 2001, of controversial British astrophysicist Fred Hoyle, an iconoclast who was known as much for his stubborn adherence to fringe beliefs as for his substantial contributions to science. Both the good and the bad in his career stemmed from the same source—a penchant for sweeping predictions based on seat-of-the-pants calculations and an intuitive sense of what must be the correct explanation according to nature’s rules.
Hoyle’s principal debate partner—from the media’s perspective at least—was Russian-Ukrainian-American physicist George Gamow. Gamow died in 1968, more than three decades before Hoyle, but their time together in the public spotlight, roughly from the early 1950s to the mid-1960s, was long enough that their battle of ideas became legendary. Their dispute concerned the origin of the universe and of the matter within it. While both agreed that space is expanding, Hoyle argued vociferously that it was infinitely old, with new matter slowly trickling into the empty space left by expansion, creating new stars and galaxies and filling the gaps over the eons. Therefore, in what he and co-creators Hermann Bondi and Thomas Gold called the “steady state universe” the cosmos essentially looks the same overall over time.
Gamow, on the other hand, believed that all matter was created in a hot, dense state, billions of years ago, when the observable universe was far smaller. During its initial fiery minutes, all of the chemical elements were forged, he believed. Along with his associates Ralph Alpher and Robert Herman, he tried to show how such a buildup might be feasible in a primordial cosmic cauldron. Dismissing the idea of a massive breach of conservation of matter and energy at the dawn of time, Hoyle derided such models (including a predecessor idea by Belgian mathematician and priest Georges Lemaître). On a BBC radio show in March 1949, he dubbed such a sudden genesis the “big bang”—a name that stuck.
Like Hoyle, Gamow often relied on his gut feelings to make scientific predictions. He had little patience for projects that required page after page of calculations and many years of effort. Thus, while their cosmological views were vastly dissimilar, their manner of conducting research had much in common.
For example, in 1928, while visiting the University of Göttingen in Germany, Gamow learned about a dilemma physicists faced in explaining the process of alpha decay, when a heavy nucleus such as uranium suddenly expels an alpha particle (a cluster of two protons and two neutrons). Clearly, the alpha particle crosses a kind of energetic barrier that normally prohibited passage, but how? Intuitively, the conundrum reminded him of a situation in quantum mechanics in which electrons had a finite chance of tunneling through a classically forbidden region.
Gamow performed a quick calculation using quantum rules and solved the alpha decay problem overnight, sharing his results with Hungarian physicist Eugene Wigner the next day. Later, Gamow learned that Princeton physicists Ronald Gurney and Edward Condon had independently developed a similar solution. Nuclear physics progressed immensely after that breakthrough. Gamow’s formula also made predictions for collisions between individual nucleons (protons and neutrons), essential for understanding how a cycle of fusion transforms hydrogen into helium in the cores of vibrant stars, generating heat and light in the process.
The buildup of chemical elements has a bottleneck, however, which took the insights of Hoyle to help unravel. Nature does not provide a simple way of creating the isotope carbon-12 and the elements above it. Big bang nucleosynthesis—the scheme developed by Gamow, Alpher and Herman to explain how the elements were forged—does not maintain a high enough temperature for a long enough time to overcome the instability of beryllium-8, one of the rungs on the ladder to reach carbon-12. Beryllium-8 decays extremely rapidly, offering it only a minuscule chance of combining with helium-4 to make carbon-12 (mathematically, the simplest way of creating that isotope), unless conditions were far more favorable than the big bang could furnish.
Because he didn’t support the big bang, Hoyle didn’t believe that the chemical elements (aside perhaps from helium) were forged in the early universe. Rather, in 1946 he brilliantly concocted an alternative method. As stars exhaust their primary source of fuel—transforming hydrogen into helium via fusion processes—their cores contract and become hotter and hotter. Such immense temperatures offer the perfect environment for element creation. Moreover, the sudden contraction at the end of a star’s lifetime is—if it is sufficiently massive—accompanied by a supernova explosion that spews the forged elements into space. In short, Hoyle’s scheme cleverly explained both the concoction and the distribution of the elements we see on Earth.
Another of Hoyle’s phenomenal insights explained how the beryllium-8 bottleneck could be overcome. He speculated that carbon-12 possessed a quantum energy level that matched up well with that of beryllium-8 combined with helium-4, making transformations at extremely high temperatures likely enough that they could happen in contracting cores. When a team of experimentalists at Caltech’s Kellogg Radiation Laboratory verified that such a carbon-12 excited state existed in nature, Hoyle’s hunch was splendidly confirmed.
The downside of the intuitive approach taken by both Hoyle and Gamow is that mere speculation might land way off the mark. In Hoyle’s case, he enjoyed intellectual fencing matches and didn’t mind if others strongly disagreed with his conjectures, as long as they remained open to debate. Hence, he clung to variations of the steady state model long after substantial evidence, starting with the discovery in the mid-1960s of a faint afterglow of radiation that permeates the universe, pointed to a hot big bang.
Moreover, in his final decades of life, he published numerous books and articles advancing fringe opinions in fields well outside his specialty. For example, he proposed that many illnesses on Earth were extraterrestrial in origin, and that a famous, well-established fossil in a London museum was a fake—without supplying credible proof of either view. Gamow did not go out on a limb in the same way. However, he often bombarded his colleagues, such as Edward Teller during their time together in the late 1930s at George Washington University, with a succession of speculative notions, most of which never went anywhere.
In short, the same kind of intuitive approach that led Hoyle and Gamow each to suggest proposals that advanced scientific understanding also steered them toward many hunches that went nowhere. Hoyle, much more so that Gamow, was known to cling to such ideas far too long. Gamow would simply move on to other subjects and schemes. Today, with many scientific enterprises much larger and far more cautious, the role of such iconoclasts is greatly diminished. Still, we might toast the boldness of mavericks in the past, such as Hoyle and Gamow, for the leaps of progress that ensued.
This is an opinion and analysis article, and the views expressed by the author or authors are not necessarily those of Scientific American.
"follow" - Google News
August 19, 2021 at 01:56AM
https://ift.tt/37Wm0W2
When Physicists Follow Their Gut - Scientific American
"follow" - Google News
https://ift.tt/35pbZ1k
https://ift.tt/35rGyU8
Bagikan Berita Ini
0 Response to "When Physicists Follow Their Gut - Scientific American"
Post a Comment